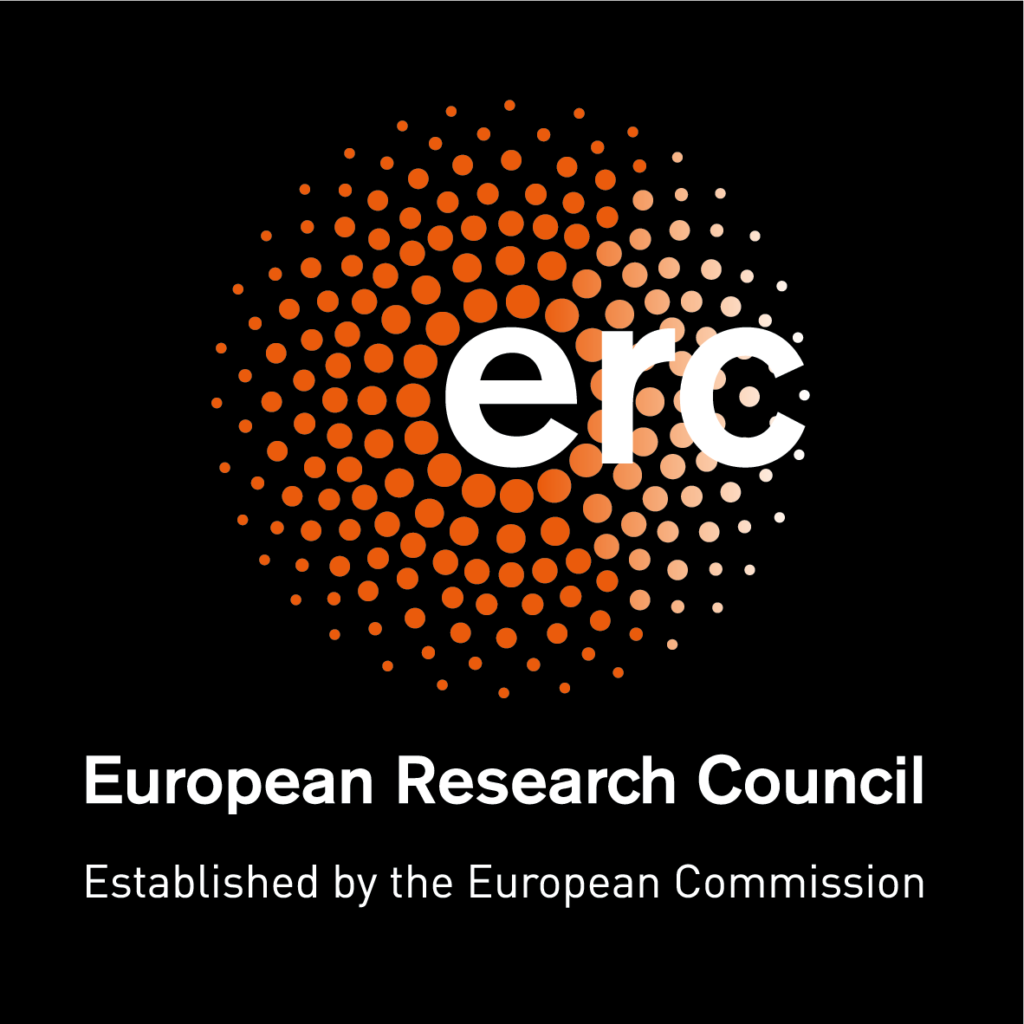
The BEC-Networks project is funded by the European Research Council (ERC) and started in February 2021. The project focuses on a complex and controllable quantum system: a network of photon Bose-Einstein condensates (BEC). This system can act as a simulator for classical spin systems, notably the XY model. When properly operated, this simulator samples the low-energy configurations of an XY model. An important goal of this project is to use photon BEC networks as a tool for solving the so-called ground-state energy problem.
The ground-state energy problem in XY spin glasses, which corresponds to finding the lowest energy state in a frustrated magnet, is a difficult combinatorial problem that is known to be NP-hard. The latter implies that every other NP problem can be mapped onto the ground state problem in XY spin glasses in polynomial time. A computer that solves this problem, thus, solves the whole class of NP problems. That includes, for example, instances of the famous traveling salesman, graph coloring, and partitioning problem. A device that significantly speeds up such calculations would represent a tremendous breakthrough in computing.
See also the press release.
Scientific output related to the objectives of this project:
#4
M. Vretenar, M. Puplauskis and J. Klaers, “Mirror Surface Nanostructuring via laser direct writing – characterization and physical origins”, Advanced Optical Materials 11, 2202820 (2023). link
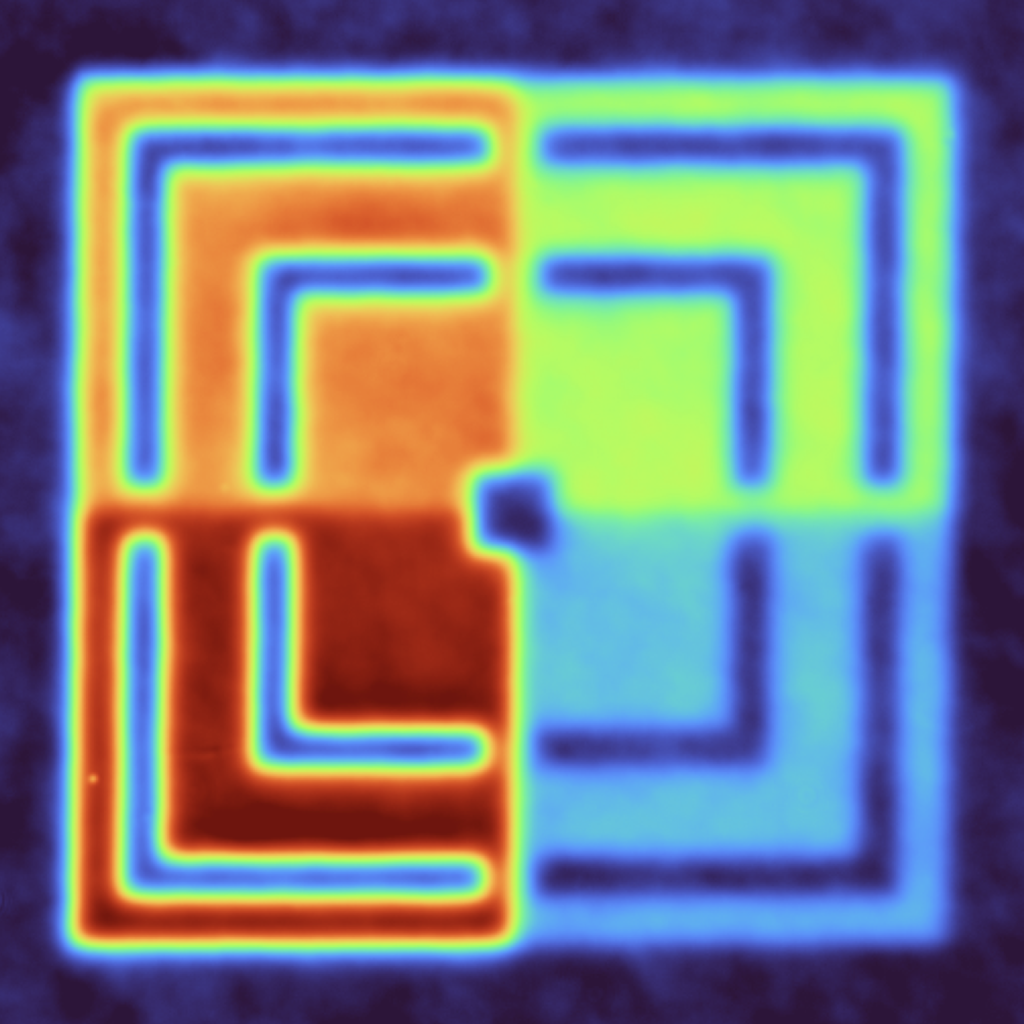
How can you control the motion of light in resonators? This is a challenge that our research group has been working on intensively for several years. Light in a resonator propagates essentially parallel to the optical axis, but can also make a small angle to it, resulting in motion in the plane of the resonator. In recent years we have developed various methods of better controlling this in-plane movement. For example, we have introduced a thermo-responsive polymer into the resonator, with which this movement can be influenced by changing the refractive index [Dung et al., Nature Photonics 11, 565 (2017)]. Complementary to this, we have also been researching how to nanostructure the surface of the mirrors, as this effectively creates a potential energy landscape for the photons that equally controls their motion.
It turns out that a highly precise nanostructuring of mirror surfaces is surprisingly easy to achieve by incorporating an absorptive layer in the dielectric stack of a mirror. By locally heating this layer with a focused laser beam it is possible to create long-term stable surface profiles with highest precision. A first publication on this method was released in 2020 [Kurtscheid et al., EPL 130, 54001 (2022)]. In the past two years, we have successively improved the technical implementation of this method and worked on a deeper understanding of the physical processes behind the nanostructuring process. The advantages of this novel type of nanostructuring of mirror surfaces can be summarized as follows: routinely achievable Angstrom-level precision, preserves highest mirror reflectivities, surface structures are long-term stable, cost-effective and fast. These properties make this technique particularly suitable for many applications in high-finesse resonators, e.g., cavity ringdown spectroscopy or interferometric precision measurements like gravitational wave detection. Wavefront shaping (particularly in the UV) also seems to be a natural application.
#3
C. Toebes, M. Vretenar and J. Klaers, “Dispersive and dissipative coupling of photon Bose-Einstein condensates”, Communications Physics 5, 59 (2022). link
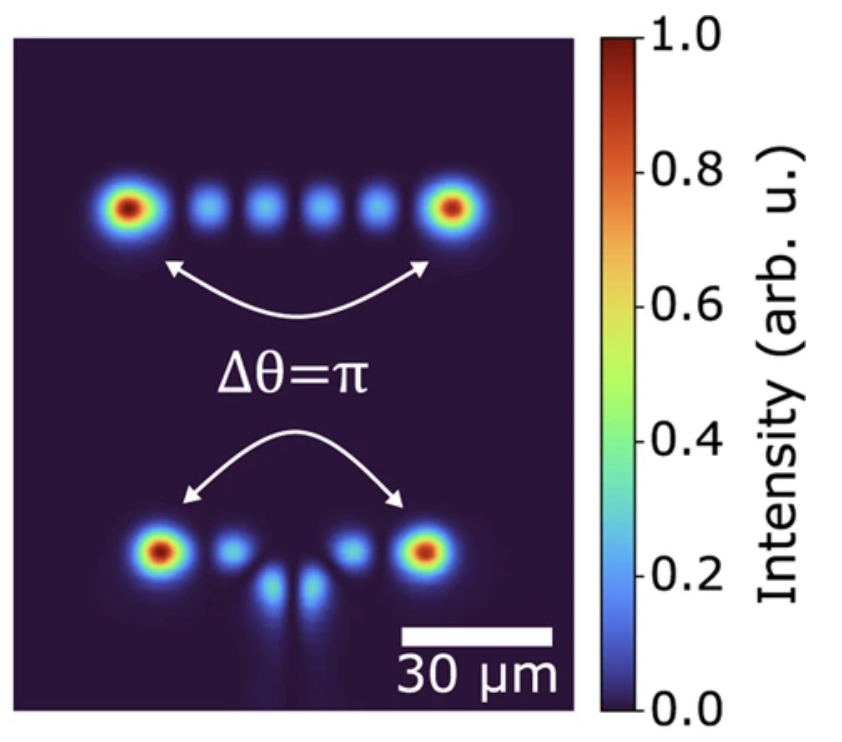
Mapping a spin model Hamiltonian to the gain function of an optical spin-glass simulator is a prerequisite for the simulator to start sampling low-energy configurations. It is furthermore desirable that the dynamics of the simulator have fixed points (including the ground state of the simulated spin model). In the photon BEC system and related platforms this is only the case if the dispersive part of the physical couplings is sufficiently small. Dissipative types of coupling, as proposed and investigated in this work, are particularly desirable in this regard.
This work is part of a collection of articles on Unconvetional computing with optical simulation of spin Hamiltonians.
#2
M. Vretenar, C. Toebes and J. Klaers, “Modified Bose-Einstein condensation in an optical quantum gas”, Nature Communications 12, 5749 (2021). link
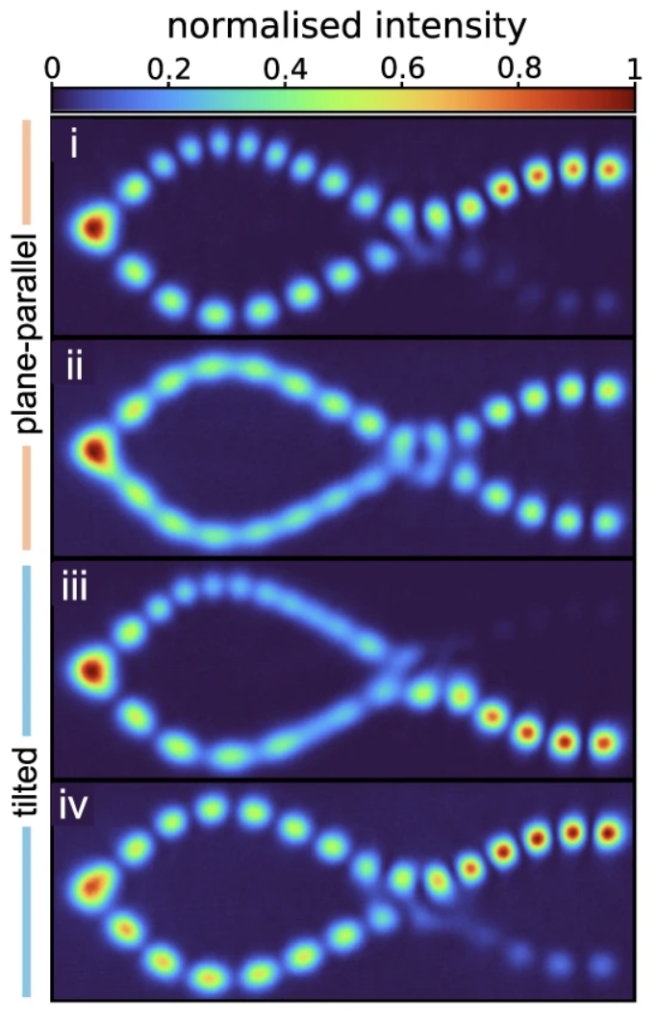
Non-equilibrium and externally controlled quantum systems are currently one of the most active research areas in physics. A main motivation is the fact that such systems are used in emerging quantum technologies such as quantum computing or quantum simulation. An equally important reason is that such systems help us gain a deeper understanding of quantum mechanics. It is often the case that the true character of a physical system only becomes apparent when it is forced to leave its “comfort zone”. One such example is superfluidity. It is only when a superfluid is accelerated from rest that its defining property, i.e., movement without dissipation becomes apparent. Another example concerns the spontaneous emission of an electronically excited atom or molecule. Only when such emitters see an environment other than the usual three-dimensional vacuum do the full mechanisms behind the spontaneous emission reveal themselves. The latter concerns, for example, the role of coherent feedback from the environment.
We also consider the Bose-Einstein condensation to be an example that fits into this list. For the case of thermal equilibrium, it is known that condensation in a Bose gas occurs in the state that minimizes the energy. This is a direct consequence of the Bose-Einstein distribution. But does that really mean that energy is the only criterion in the formation of a Bose-Einstein condensate? In our work, we investigate the Bose-Einstein condensation of a two-dimensional photon gas in an environment with controlled dissipation and feedback realized by a potential landscape that effectively acts as a Mach-Zehnder interferometer. Our measurements offer a highly systematic picture of Bose-Einstein condensation under non-equilibrium conditions. We show that by adjusting their frequency, Bose-Einstein condensates naturally seek to minimize particle loss and destructive interference in their environment. This hitherto unknown ability remains hidden in thermal equilibrium but becomes visible when the condensate is forced to exchange particles with its environment under non-equilibrium conditions. Beyond a deeper understanding of Bose-Einstein condensation, our findings open new pathways for quantum simulations with optical Bose-Einstein condensates.
See also the press release.
#1
M. Vretenar, B. Kassenberg, S. Bissesar, C. Toebes and J. Klaers, “Controllable Josephson junction for photon Bose-Einstein condensates”, Physical Review Research 3, 023167 (2021). link
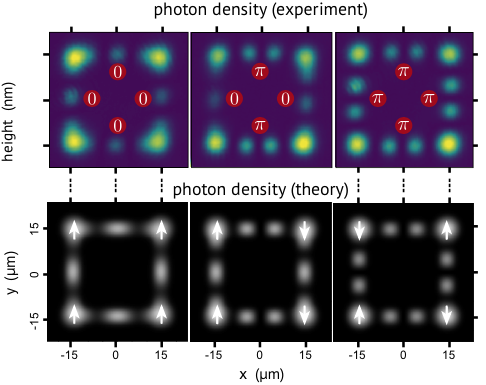
A long-term goal of our research is to open up photon Bose-Einstein condensation in lattice systems for novel optical computation schemes such as analog spin glass simulation and oscillatory neural networks. To achieve this, two technological challenges must be overcome. The first challenge concerns the creation of attractive and repulsive potentials for the photon gas inside a microresonator. This allows us, in particular, to confine photons to periodic lattice potentials.
The second challenge concerns the control of the tunnel couplings between lattice sites. This challenge is addressed in our paper published in Physical Review Research. In this work, we demonstrate controllable phase relations between two photon Bose-Einstein condensates. By changing the index of refraction between lattice sites, we can realize both in-phase and anti-phase coupling of two neighboring condensates. The scheme, which we propose and investigate in our publication, can be regarded as an optical analogue of a controllable Josephson junction. Similar to a transistor for electronics, a controllable Josephson junction is a crucial prerequisite for all-optical analog spin glass simulation and oscillatory neural networks.